- Radioactive Isotope Of Hydrogen Symbol
- Radioactive Isotope Of Hydrogen Vs
- Radioactive Isotopes Examples
- The Radioactive Isotope Of Hydrogen Is
- Uses Of Hydrogen Isotopes
The most stable radioactive isotope is tritium, with a half-life of 12.32 years. Hence, option B is correct. (Recall that tritium is a radioactive isotope of hydrogen.) Tracers can also be used to follow the steps of a complex chemical reaction. After incorporating radioactive atoms into reactant molecules, scientists can track where the atoms go by following their radioactivity. Isotopes of an element have the same atomic number but different mass numbers. Hydrogen is the first element present in the periodic table and has one proton. Hydrogen has three isotopes protium, deuterium, and tritium. The three isotopes are different because of the difference in the number of neutrons present in them. Radioactive Tritium is a radioactive nuclide with a half-life period of 12.4 years and emits low energy β particles. Schematic representation of isotopes of hydrogen is as follows.
Summary
Nuclear facilities emit very large amounts of tritium, 3H, the radioactive isotope of hydrogen. Much evidence from cell/animal studies and radiation biology theory indicates that tritium is more hazardous than gamma rays and most X-rays. However the International Commission on Radiological Protection (ICRP) continues to underestimate tritium’s hazard by recommending a radiation weighting factor (wR) of unity for tritium’s beta particle emissions. Tritium’s exceptionally high molecular exchange rate with hydrogen atoms on adjacent molecules makes it extremely mobile in the environment. This plus the fact that the most common form of tritium is water, ie radioactive water, means that, when tritium is emitted from nuclear facilities, it rapidly contaminates all biota in adjacent areas. Tritium binds with organic matter to form organically bound tritium (OBT) with long residence times in tissues and organs making it more radiotoxic than tritiated water (HTO). Epidemiology studies indicate increases in cancers and congenital malformations near nuclear facilities. It is recommended that nuclear operators and scientists should be properly informed about tritium’s hazards; that tritium’s safety factors should be strengthened; and that a hazard scheme for common radionuclides be established.
Contents
- Overview
- The Multiple Hazards of Tritium
- Molecular Exchange
- How are we exposed to tritium?
- Organically Bound Tritium
- Longevity of OBT in the Environment
- How do we estimate tritium risks?
- Epidemiology
- The Abuse of Statistical Significance Tests
- Recommendations
- References
1.Overview
This report summarises current understandings of the biological and health effects of exposures to tritium and comments on the risks faced by people living near nuclear facilities. It is mainly based on previous work on Canadian nuclear facilities because of the availability of Canadian data. However the conclusions are broadly applicable to all nuclear facilities, as concentrations are discussed rather than simple amounts.
Tritium is the radioactive isotope of hydrogen with a half life of 12.3 years. It decays through the emission of a beta particle with a maximum energy of 18.7 keV and an average energy of 5.7 keV (Okada, 1993). The mean free path of tritium’s decay beta particle in tissue is about 0.6 µm, the diameter of a human chromosome. It exists in nature mainly as elemental tritium (HT) and as radioactive water (HTO). The biological half-life of HTO in humans is about 10 days but this can be shortened by forced diuresis. The biological half-lives of OBT depend on the atom (eg C, N, and P) to which the tritium is bound and the longevity of its organic molecule, eg DNA and RNA are strongly conserved. In areas remote from nuclear facilities, background levels of tritium in water are about two to five becquerels (Bq) per litre mainly as the residue from atmospheric atomic bomb tests in the 1950s and 1960s.
In the latter half of the 20th century, tritium was often regarded as a “weak” nuclide. This attitude changed after the publication of the CERRIE report on internal emitters in 2004, as tritium is the most commonly experienced internal emitter. The CERRIE report resulted in major reports on tritium by radiation safety agencies in the UK (AGIR, 2008), Canada (CNSC, 2010a; 2010b) and France (ASN, 2010). In addition, the French Institute de Radioprotection et de Sûreté Nucléaire published six reports on tritium (IRSN, 2010a; 2010b; 2010c; 2010d; 2010e; 2010f). In particular, these reports noted that tritium exposures resulted in internal radiation doses which were difficult to estimate and contained large, possibly very large, uncertainties which could render them unreliablThe most comprehensive report on tritium was published by the UK Government’s senior Advisory Group on Ionising Radiation (AGIR, 2008). This strongly recommended that tritium’s hazard (ie, its Relative Biological Effectiveness or RBE) should be doubled. A draft report by the US EPA (2006) recommended its RBE should be increased by a factor of 2.5. Other scientists (Fairlie, 2008; Fairlie, 2007a; Fairlie, 2007b; Melintescu et al, 2007; Makhijani et al, 2006) have presented evidence for even larger increases in tritium’s radiotoxicity.
The above reports drew attention to tritium’s various properties which mark it out as an unusually hazardous radionuclide. These include
- its relatively long half-life of 12.3 years,
- its mobility and cycling as radioactive water in the biosphere,
- its multiple pathways to man,
- its ability to swap instantaneously with H atoms in adjacent materials,
- its comparatively high relative biological effectiveness (RBE) of 2 to 3,
- its propensity to bind with cell constituents to form organically-bound tritium (OBT) with heterogeneous distribution in humans, and
- the short range of its beta particle meaning that its damage depends crucially on its location within cellular molecules, including DNA
- in its oxide form, tritium is generally not detected by commonly-used survey instruments (Okada et al, 1993), and
- in its elemental form, tritium diffuses through most containers, including those made of steel, aluminium, concrete and plastic. See for example, https://www.ianfairlie.org/news/continued-radioactive-emissions-from-old-closed-nuclear-reactors/
In sum, tritium presents numerous challenges to conventional dosimetry and health risk assessment. However several very recent reports have indicated little awareness of these problems with tritium.
Molecular Exchange
Many reports distinguish between elemental tritium (HT) and tritiated water vapour (HTO) emissions. However in the environment, tritium atoms rapidly exchange with the stable H atoms in water (and water vapour) through the phenomenon of molecular exchange. Therefore all tritium releases should be treated as HTO. This is common practice in industry reports (eg Davis et al, 1997). This quick transfer mechanism is important as HTO is more radiotoxic than HT. In terms of Annual Limits of Intake, HTO in air is considered 25,000 times more hazardous than HT (ICRP, 1979), partly because the body avidly takes up water but not hydrogen gas.
To explain this in more detail, in matter, all atoms engage in exchange reactions to varying degrees with like atoms in adjacent molecules. This means that tritium atoms in HT or HTO swap positions with stable H atoms in the environment in the hydrosphere and in biota, including humans. H and T, the smallest atoms are prominent as regards exchange reactions. These exchange reactions are very quick, taking about 10-15 seconds on average.
As the most common hydrogen-containing material in the environment is water itself tritium in HT emissions very quickly becomes HTO. In practical terms, open water surfaces and biota downwind, including all plants, animals and humans, would become contaminated with tritium up to the level of the tritium concentration in the atmosphere. For example, it would include vegetables and fruit in market stalls and shops (Inoue, 1993)
How are we exposed to tritium?
Let’s examine what actually happens when tritium is emitted from nuclear facilities whether as water vapour or as elemental tritium. It travels via multiple environmental pathways (vapour plumes, rivers, crops etc) to reach humans. Human intakes occur via
- skin absorption,
- inhalation of contaminated water vapour, and
- ingestion of contaminated food and wate
When tritium enters the body, it is readily taken up through exchange mechanisms by metabolic reactions and by cellular growth. Over 60 percent of the body’s atoms are hydrogen atoms, and every day about five per cent of these are egaged in metabolic reactions and cell proliferation. The result is that a proportion of the tritium taken in is fixed to lipids, carbohydrates, proteins and nucleoproteins such as DNA and RNA. This is organically bound tritium (OBT) and is discussed furtherbelow.
These unusual properties suggest that tritium should be regarded as hazardous by radiation protection authorities. Unfortunately this is not the case. Tritium’s unusual properties are not at all recognised by the ICRP and national authorities which take their lead from the ICRP. Unfortunately, no international hazard index for radionuclides exists at present although one has been proposed (Kirchner, 1990).
Another controversy exists over the ICRP’s continued recommendation of a radiation weighting factor (wR) for tritium of 1 despite copious evidence (Fairlie, 2007a) that it should be doubled or trebled. This debate has lasted for more than sixty years.

It should be borne in mind that the ICRP, despite its title, is not an official body but a voluntary one. In the past, its scientific interpretations have been criticised (eg Shrader-Frechette, 1985). Although it has adopted a more open appearance in recent years, when it comes to crunch issues such as collective dose, internal dosimetry, and the RBE for tritium, it remains principally concerned with protecting the interests of its members rather than those of the general public. It appears that non-scientific considerations play a part in the ICRP’s policies on tritium, particularly as regards nuclear weapons production plants in the past, nuclear power facilities at present and proposed fusion facilities in future.
Organically Bound Tritium (OBT)
OBT is non-uniformly distributed and is retained for longer periods than tritiated water. ICRP dosimetric models for tritium assume the opposite – that tritium is homogenously distributed in the tissues/organs as tritiated water (HTO) and is quickly excreted. The problem is that exposures from OBT are generally higher than those from HTO. The longer people are exposed to tritiated water emissions, the higher their levels of OBT become until, in the case of exposures lasting years, equilibria are established between HTO and OBT levels. Again ICRP dosimetric models assume the opposite: only single exposures are considered so that their estimates of OBT levels remain low.
Organically bound tritium (OBT) is detectable in most organic materials such as plants, animals and soils near nuclear facilities. The non-exchangeable form of organically bound tritium, ie 3H bound to carbon atoms, is mainly produced by photosynthesis in plants and by metabolic processes in animals. A second form of OBT called exchangeable OBT is more loosely bound to P, N and S atoms.
In more detail, the behaviour of OBT (both forms) in the environment is not well understood as it is very heterogeneously distributed in natural ecosystems. Nevertheless, OBT has been recognized as more significant than tritiated water in understanding tritium’s behaviour (Kim et al, 2013) partly because OBT measurements provide a more accurate representation of tritium in the environment due to its longer retention time than HTO (Kim and Roche, 2012).OBT can be incorporated into all biochemical compounds, including amino acids, sugars, starches, lipids and cell structural materials. It therefore has longer retention times than tritiated water which has a biological half-life of only about 10 days in adults. Some biomolecules are very long-lived indeed, e.g. phospholipids in nerve cells and the DNA and RNA macromolecules which can last for lifetimes. These longer retention times result in OBT’s greater radiotoxicity than tritiated water. The ICRP has recommended an OBT ingestion exposure coefficient 2.3 times greater than that for HTO. (ICRP dose coefficients for adults are 1.8 x 10?11 Sv/Bq for tritiated water and 4.2 x 10?11Sv/Bq for OBT.) However evidence suggests it should be at least 5 times greater (Fairlie, 2008).
Following a single HTO intake, the current ICRP model assumes 3% is bound as OBT and may be neglected. But Trivedi et al (1997) estimated that up to 9% is bound as OBT in humans. Animal studies also indicate that OBT levels must be considered, as it is cleared from the body much more slowly than HTO. Commerford et al (1982) found, after a transient HTO exposure, tritium remained bound to mouse DNA and histone up to eight weeks later. They concluded that the OBT doses would exceed HTO doses overall even from a single administration.
The same goes for chronic HTO intakes. Commerford, Carsten and Cronkite (1977) found most of the tritium dose came from OBT two to three days after stopping chronic HTO administration to mice. Similarly, Rogers (1992) concluded OBT was the principal determinant in tritium doses to mice following chronic HTO exposures. More recently, Kim et al (2013a) discussed the OBT contribution to tritium exposures from chronic tritium releases to air. They compared 11 studies whose mean OBT contribution to total tritium exposures was 21%. In other words, estimates of HTO exposures from nuclear facility emissions should be increased by a factor of 1.25.
Longevity of OBT in the Environment
Eyrolle-Boyer et al (2014) have suggested that raised OBT levels can persist in the environment for several decades following HTO releases. They found that terrestrial biomass pools which had been contaminated by the global atmospheric fallout of tritium from nuclear weapons testing in the 1950s and 1960s constituted a significant delayed source of OBT, resulting in an apparent increase in OBT levels compared to HTO levels. This finding explains OBT/HTO ratios greater than 1 observed in areas not affected by radioactive releases. This finding also supports the findings by Ichimasa (1995) of long-term raised OBT levels near the Chalk River nuclear plant in Canada following chronic tritium releases.
Thompson et al (2015) stated that, as soil acts as a repository for decaying organic matter, OBT concentrations in soil represent long-term reservoirs of past tritium releases. It added “Our data support the mounting evidence suggesting that some parameters used in environmental transfer models approved for regulatory assessments should be revisited to better account for the behaviour of HTO and OBT in the environment and to ensure that modelled estimates (e.g. plant OBT) are appropriately conservative.” Unfortunately this advice does not appear to have been heeded in most countries.
How do we estimate tritium risks?
In the assessment of risks from tritium releases, aerial emissions are more important than liquid discharges for two reasons. First, the key parameter in estimating radiation doses to local people is the nuclide concentration in environmental materials. Contrary to what many think, air emissions result in higher environmental concentrations than water discharges. The reason is dilution: a cubic metre of water contains a million grams which dilutes radioactive contaminants far more effectively than a cubic metre of air with a water content of ~10 grams (Davis et al, 1996) ie > 100,000 times more. This is not to accept that dilution is a solution to pollution. It merely reflects existing (ill-advised) methods of disposing nuclear wastes. Second, individual and collective doses from air emissions are much larger than from discharges to water. Accordingly this report deals mainly with air emissions.
To assess risks, the official approach is to estimate tritium’s radiation doses as expressed in Sv units, but severe problems exist with tritium’s dosimetry – (Fairlie, 2007a,b,c; Fairlie, 2008). Estimates of internal doses and risks arising from exposures to internal emitters such as tritium are considered to be unreliable – see the conclusions of the CERRIE Report (2004).
When assessing risks, it is considered preferable to use radioactivity instead of radiation, in other words we should use tritium’s Bq emissions and intakes and concentrations instead of Sv doses. At least, radioactivity can be physically measured whereas radiation doses are estimates. The radioactivity approach has been used by other scientists (eg Osborne, 2002).
Air concentrations of tritium will vary considerably over time as spikes in tritium emissions regularly occur. Pulsed tritium emissions could result in heavy labelling of cells being formed in the embryos and fetuses of nearby pregnant women at that particular moment. This fear was expressed by Professor Edward Radford in his 1979 testimony to the Ontario Government’s Select Committee on Ontario Hydro Affairs: Hearings on The Safety of Ontario’s Nuclear Reactors, July 10 1979. See http://www.ccnr.org/tritium_2.html#scoha].This provides the basic mechanism for the hypothesis explaining the large observed increases in leukemia in subsequent children born near nuclear reactors (Fairlie, 2014).
Local people living near nuclear facilities can be exposed to tritium via
- drinking tritiated liquids, and ingesting foodstuffs contaminated with tritiated water vapour, e.g. from local markets and fruit stalls
- inhaling tritium gas and tritiated water vapour, and
- skin absorption of tritiated water vapour
In fact, local people could have high intakes of tritium, so that, ideally, their tritium concentrations should be measured using urine analyses for HTO and non-invasive bioassays, such as nail clippings and hair clippings, for OBT. Unfortunately this is almost never done among members of the public.
Yardstick for tritium in drinking water
To use radioactivity (measured in Bq) as a measure of risk, we need a yardstick for safe levels in drinking water, which is constructed as follows.
An annual risk of one in a million (10-6) of fatal cancer is considered acceptable (HSE, 1988). Using this risk level, the Ontario Government’s Ontario Drinking Water Advisory Council (ODWAC, 2009) recommended a maximum concentration of 20 Bq/L for tritium in drinking water. If we multiply this concentration by Health Canada’s average annual water intake of 550 litres for adults, we get~10,000 Bq of tritiated water per year or 30 Bq per day for adults, correct to one significant figure. This may be used as a rough yardstick for an acceptable annual intake of tritium for adults.
This yardstick very much depends on the value chosen for the drinking water limit, and different views exist on this: table 1 shows various limits in play. It is reasonable to use the official limit recommended by the Ontario Government’s ODWAC – ie 20 Bq/L.
Table 1.Tritium Concentration Limits in drinking water – Bq per litre | ||
AGENCY | DATE | TRITIUM LIMIT BQ PER LITRE |
Ontario Government’s Advisory Committee on Environmental Standards | 1994 | 20* |
EC (European Commission,1998) | 1998 | 100 |
US State of Colorado target | 2008 | 18 |
US State of California target | 2008 | 15 |
Ontario Government (ODWAC,2009) | 2009 | 20* |
CNSC design guide for groundwater (CNSC,2011) | 2011 | 100 |
* after an initial period of 100 Bq/L. |
Epidemiological Evidence of RisksBecause of methodological limitations, epidemiology studies are a blunt tool for discovering whether adverse effects result from radiation exposures. These limitations include:
- under-ascertainment, i.e., people move away, or cases are not found or reported.
- strict data requirements: ideally, epidemiology data is required with good case identification, uniform registration, clear diagnostic criteria and uniformity of data collation. These data requirements are often difficult to fulfil and make large demands on time and resources.
- confounding factors: the true causes of morbidity or mortality can be uncertain due to confounding factors such as socio-economic status and competing causes of death.
- bias: smoking and alcohol cause major increases in overall mortality and morbidity, and in cancer and cardiovascular disease. These require careful handling of the raw data to avoid bias.
- poor signal to noise: only large, expensive and lengthy epidemiology studies are able to reveal effects where the signal (added cancers) is weak, and the noise (large numbers of spontaneous cancers) is strong.
- uncertain doses: establishing causality often requires estimating doses in order to show a dose-effect relationship. However, large uncertainties often exist in estimating doses – especially from internal radiation, e.g. from tritium.
- wide confidence intervals: usually findings (e.g. risks or odds ratios) are expressed with 95% confidence intervals, that is, the range of values within which the true value lies within 95% of the time. But often this range can be very wide – simply because of low numbers of cases. This can severely limit what we can conclude from the findings.
Many epidemiology studies are ecologic studies, that is, quick inexpensive studies which look at health statistics in tables and notate individual data. Their findings are usually regarded as indicative, but not conclusive. If their findings suggest an adverse effect then these should be investigated further by more detailed cohort or case-control studies. The latter match “cases” (i.e. those with an adverse health effect) with randomly-selected similar individuals without an adverse effect, in order to minimise under-ascertainment. However few of these are actually carried out because of their expense and long time-spans. Sometimes they are not carried out for political reasons because findings of increased cancers are not welcome.
A disconcerting finding is that a substantial number of epi studies near NPPs conclude there are no findings of ill health even though positive increases were in fact observed. That is, the researchers were unable to accept the evidence of their own work. It is difficult to comment on this cognitive dissonance (few studies seem to exist on this phenomenon) but it is apparently often due to unacknowledged biases or to group-think re the impossibility for ill-health effects to exist near nuclear facilities. In their conclusions, such authors have discounted their findings using a variety of reasons including
- the results were too wide-ranging,
- too many comparisons were being made, ie some were due to chance alone
- lack of a consistent results (eg some cancer types observed but not other types)
- lack of a trend showing increased risks rising with doses,
- lack of a distance trend, and
- lack of statistical significance in observed increased risks – see next section
However there is a serious problem here. If similarly increased health effects had been observed near, say, a lead smelting factory or an asbestos mine, would they be dismissed by referring to these rationales? I rather doubt it. In other words, what is occurring here is that hidden biases in favour of nuclear power are in play. In my view, such conflicts of bias should be declared at the outset just as conflicts of interest are nowadays.
The Abuse of Statistical Significance Tests
Many epi studies of cancer near NPPs have found increased risks but dismissed them as not “statistically significant”. This wording often misleads lay readers into thinking that a reported increase is unimportant or irrelevant. But, in statistics, the adjective “significant” is a specialist word used to convey a narrow meaning, ie that the likelihood of an observation being a fluke is less than 5% (assuming a p = 5% test were used). It does not mean important or relevant.
Also this phrase is usually employed without explaining that the chosen significance level is quite arbitrary. There is no scientific justification for using a 5% level or any other test level: it is merely a matter of convenience. In other words, it is quite possible for results which are “not significant” when a 5% test is applied, could become “significant” when a 10% or other test level were used.
The existence of this practice has historical parallels. In the 1950s, dozens of health studies financed by tobacco companies acted to sow seeds of doubt about the health effects of cigarette smoking for many years. The use of statistical significance was a common stratagem in these studies. as described in US books, see here and here. Similarly, pharmaceutical companies have been shown to run trials on their own drugs designed to minimise their side effects. See here. Again the lack of statistical significance was used as a ploy in these trials.However these bad practices may soon have to stop. In March 2019, the journal Nature published an important editorial “It’s time to talk about ditching statistical significance” which argued against the use of statistical tests in health studies. The same edition contained a commentary “Scientists rise up against statistical significance” signed by 853 scientists worldwide, with about 80 in the UK. It called for call for an end to, inter alia, “the dismissal of possibly crucial effects” in health studies through the inappropriate use of statistical testing. In the US, it reported that the American Statistical Association (ASA) had published a scientific article with the same aim. See https://www.ianfairlie.org/news/uk-and-us-scientists-call-for-statistical-significance-tests-to-be-dropped-in-health-studies/
The Nature editorial stated that statistical tests will continue to be needed in some industrial applications where a yes/no decision is needed, but crucially not in health research, ie epidemiology studies and clinical trials. Why? Because their use in health studies can be biased due to ulterior motives or be insufficiently nuanced.
The Nature article explained many health researchers in the past had “chucked their results into a bin marked ‘not significant’ without further thought”. Instead researchers should have considered matters such as “background evidence, study design, data quality and an understanding of underlying mechanisms, as these are often more important than p values or confidence intervals”. In particular, they should have discussed the health implications of their non-statistically significant findings.
The misuse of statistical significance is an important issue for four reasons. First, because the use of statistical significance tests has often led to the wrong result, especially in clinical trials, and the same is true in epidemiology studies in my experience. Several authrs have reported that the rejection of findings for significance reasons can often hide real risks (Axelson, 2004; Whitley and Ball, 2002).
Second, as Nature states “the rigid focus on statistical significance encourages researchers to choose data and methods that .. .yield statistical non-significance for an undesired result, such as potential side effects of drugs — thereby invalidating conclusions.” This damning verdict applies with equal force to the undesired result of observed increases in health effects in an epidemiology study. For decades, some scientists, sadly including those employed at UK government agencies, have dismissed risk findings in epidemiology studies near nuclear facilities by concluding they showed no “significant” raised risks or that excess risks were “not significant”, or similar phrases.
A third reason also mentioned in the Nature article, is that we must re-examine past studies which used lack of statistical significance to dismiss observed increases as these conclusions are now unreliable. This verdict applies, for example, to past studies by the UK Government’s Committee on the Medical Aspects of Radiation in the Environment (COMARE) studies which observed leukemia increases near UK nuclear facilities but dismissed them because they were not statistically significant. These include, for example,
COMARE (2011) Committee on Medical Aspects of Radiation in the Environment Fourteenth Report. Further Consideration of the Incidence of Childhood Leukaemia Around Nuclear Power Plants in Great Britain. HMSO: London.
COMARE (2016) Committee on Medical Aspects of Radiation in the Environment (COMARE) Seventeenth Report. Further consideration of the incidence of cancers around the nuclear installations at Sellafield and Dounreay HMSO: London.
The fourth reason is the vital factor of size in epidemiological studies, ie the numbers of observed cases of ill effects in a population. This is because the probability (ie p-value) that an observed effect may be a fluke is affected by both the magnitude of effect and the size of study (Whitely and Ball 2002; Sterne and Smith, 2001). If the study size is small, its findings often will not be statistically significant regardless of the presence of the adverse effect (Everett et al, 1998).
Conclusion
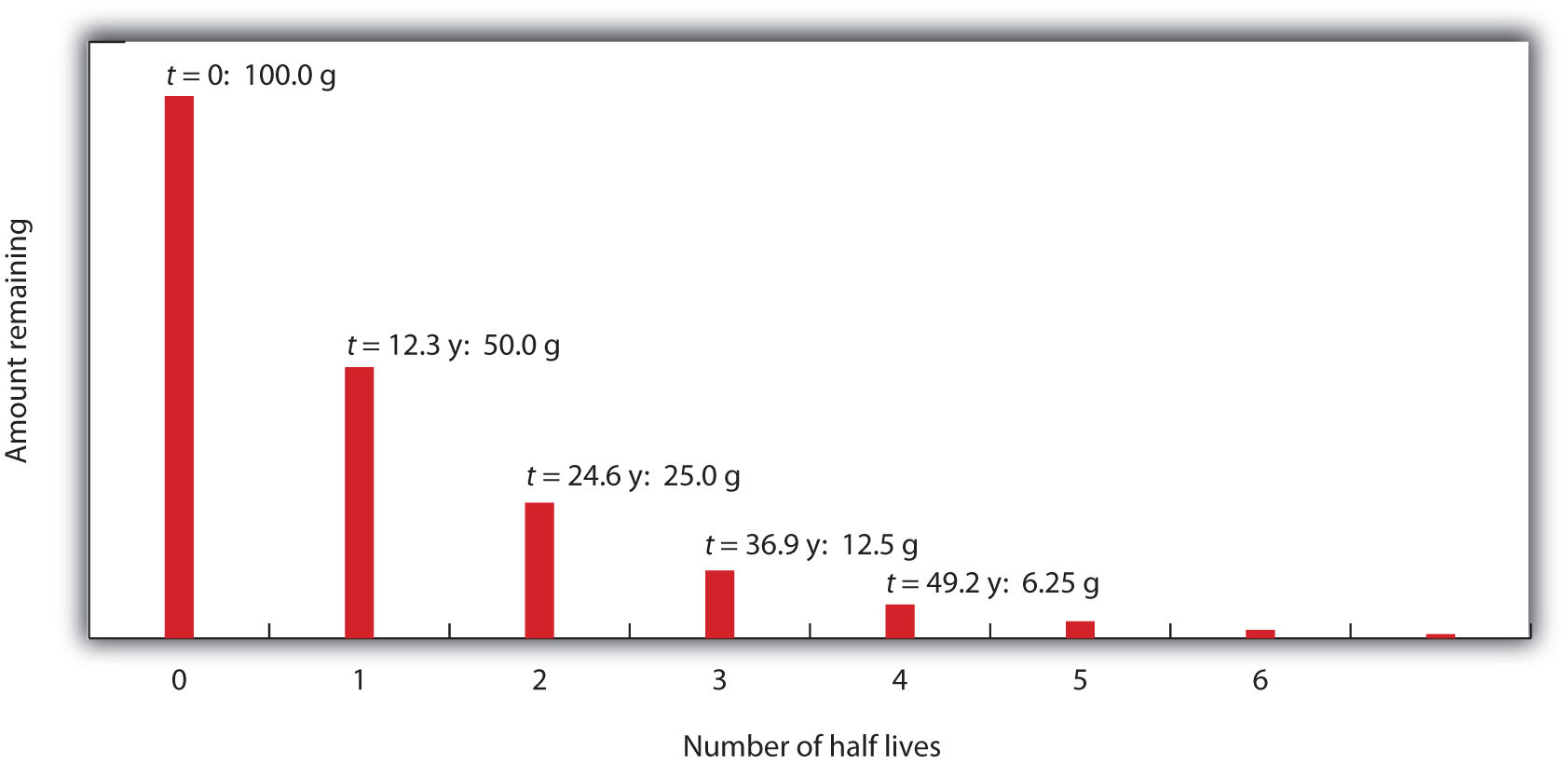
I have argued that tests for statistical significance have been misused in epidemiological studies on cancers near nuclear facilities. These in the past have often concluded that such effects do not occur or they downplayed any effects which did occur. In fact, copious evidence exists throughout the world – over 60 studies – of raised cancer levels near NPPs. This is discussed in my scientific article in 2014 on a hypothesis to explain cancers near NPPs. Most (>75%) of these studies found cancer increases but because they were small, their findings were often dismissed as not statistically significant. In other words, they were chucked in the bin marked “not significant” without further consideration. I conclude by asking open-minded scientists and observers to reconsider their views about the above 60+ studies and the misleading COMARE reports showing raised cancer levels near NPPs. Just as people were misled about tobacco smoking in previous decades, perhaps we are being misled about raised cancers near NPPs nowadays.
Recommendations
It is recommended that
- a limit of 20 becquerels per litre (Bq/L) for drinking water
- Urine tests and non-invasive bioassay tests should be carried out on volunteers living nearby to ascertain HTO/OBT levels.
- Local residents should be advised to avoid consuming locally-grown foods and water from local wells.
- Local women near NPPs intending to have a family, and families with babies and young children should consider moving elsewhere. It is recognised this recommendation may cause concern but it is better to be aware of the risks to babies and young children than be ignorant of them.
- Employees and their spouses, especially young workers, should be informed about the hazards of tritium.
- Statistical significance in epi study findings should not be arbitrarily used to dismiss positive findings.
- A hazard index of radionuclides should be established.
References
AGIR. Review of risks from tritium. Documents of the Health Protection Agency: Radiation, Chemical and Environmental Hazards, REC-4. November 2007. http://www.hpa.org.uk/web/HPAweb&HPAwebStandard/HPAweb_C/1197382220012
Altman DG and Bland JM (1995) Absence of evidence is not evidence of absence. BMJ 311 pp 485.
ASN (2010) White Paper on Tritium. Autorite de SecuriteNucleaire (French Nuclear Safety Authority).Paris France.http://livre-blanc-tritium.asn.fr/plus/telechargements.html
Boyd MA (2009) The Confusing World of Radiation Dosimetry. WM 2009 Conference, March 1-5, 2009, Phoenix, AZ 9444 M.A. Boyd http://www.wmsym.org/archives/2009/pdfs/9444.pdf
CERRIE Report of the Committee Examining Radiation Risks of Internal Emitters. Chilton, Didcot: National Radiological Protection Board; 2004. Available at: http://www.cerrie.org
CNSC (2008) Standards and Guidelines for Tritium in Drinking Water. Part of the Tritium Studies Project. INFO 0766.
CNSC (2010) Health Effects, Dosimetry and Radiological Protection of Tritium.Canadian Nuclear Safety Commission.INFO-0799. Ottawa, Canada.
CNSC (2011) Tritium Studies Project Synthesis Report INFO- 0800 January 2011
Cox R, Menzel H-G, Preston J. Internal dosimetry and tritium – the ICRP position. J Radiol Prot. 2008; 28: 131-135.:http://www.iop.org/EJ/article/0952-4746/28/2/E02/jrp8_2_e02.pdf?request-id=af51e9d4-3bcc-4a5b-a878-7ebb2fcad86d
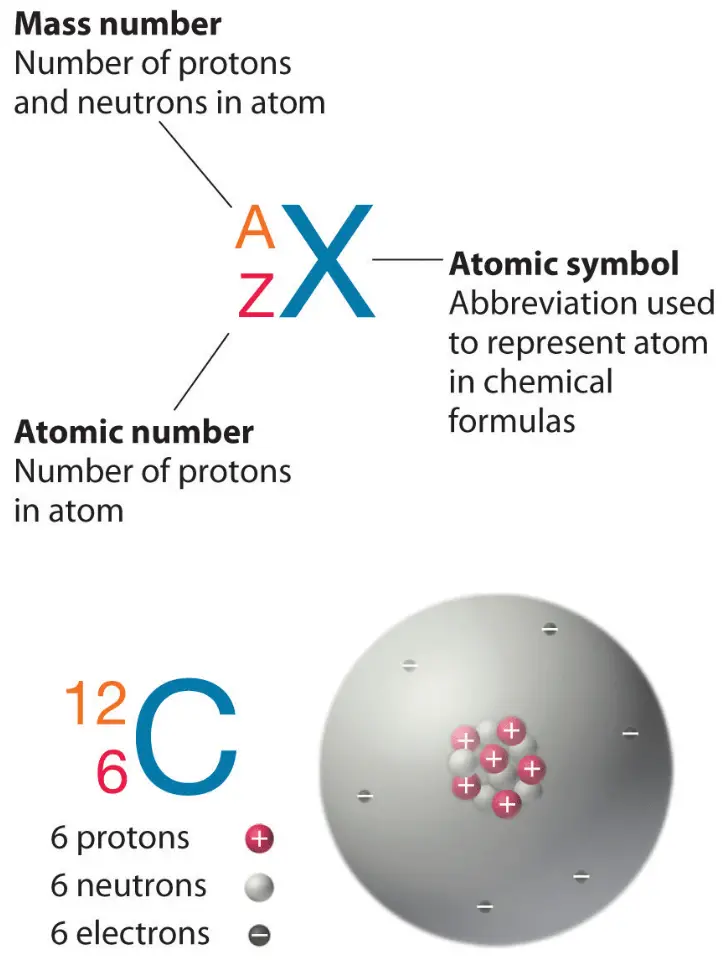
Coyle PE. Laser fusion: status, future and tritium control. In: Behaviour of tritium in the environment. Proceedings of a Symposium. San Francisco: International Atomic Energy Agency and Organisation for Economic Co-operation and Development Nuclear Energy Agency. 1978.
Radioactive Isotope Of Hydrogen Symbol
Davis PA, Amiro BD, Workman WJG, and Corbett BJ (1996) HTO Transfer from Contaminated Surfaces to the Atmosphere: A Database for Model Validation. AECL-11222.)
Davis PA, Peterson SR, Amiro, BD (1997) Revision of UNSCEAR document “Dose assessment methodologies for tritium and radiocarbon.” Chalk River, Canada: Atomic Energy of Canada Limited; Report RC-M-27.
Durham Region Health Department (2007), Radiation and Health in Durham Region 2007. Whitby, Ontario: The Regional Municipality of Durham. http://www.durham.ca/departments/health/health_statistics/radiationHealthReport2007.pdf
Radioactive Isotope Of Hydrogen Vs
Edwards R (2007) Tritium hazard rating should be doubled. New Scientist. 29 November 2007. Available at: http://www.newscientist.com/article/dn12984-tritium-hazard-rating-should-be-doubled.html
Environmental Protection Agency. Modifying EPA radiation risk models based on BEIR VII (draft White Paper). Washington DC: EPA, 1 August 2006: 27 – 28.
European Commission (1998)Council Directive 98/83/EC on the quality of water intended for human consumption. Official Journal of European Community L330: pp 32-54.
Fairlie I (2005) Uncertainties in Doses and Risks from Internal Radiation.Medicine, Conflict and Survival2005, 21(2):111-126.
Fairlie I (2007a) RBE and wR values of Auger emitters and low-range beta emitters with particular reference to tritium. Journal of Radiological Protection 27:157-168.
Fairlie I (2007b) Tritium Hazard Report: pollution and radiation risk from Canadian nuclear facilities. Greenpeace Canada. June 2007.
Fairlie I. (2007c) Tritium hazard report: pollution and radiation risk from Canadian nuclear facilities. Greenpeace Canada. June 2007. Available from: http://www.greenpeace.org/raw/content/canada/en/documents-and-links/publications/tritium-hazard-report-pollu.pdf
Fairlie I (2008) The hazards of tritium revisited. Medicine, Conflict and Survival. Vol 24:4. October 2008. pp 306 -319. http://www.informaworld.com/smpp/content~content=a904743144~db=all~order=page
Fairlie I (2009) “Childhood Cancers near German Nuclear Power Stations: hypothesis to explain the cancer increases”. Medicine, Conflict and Survival Vol 25, No 3, pp206–220.
Fairlie I(2008) New evidence of childhood leukaemias near nuclear power stations. Med Confl Surviv.2008; 24:219-227.
Fairlie I (2014) A hypothesis to explain childhood cancers near nuclear power plants J Environ Radioact. 133 (2014) pp 10- 17.
Health Canada (1994) Human Health Risk Assessment for Priority Substances. Ottawa, Canada: Ministry of Supply and Services, Canada.
Health Canada (2001) Environmental Radioactivity in Canada.Radiological Monitoring Report.Ottawa Canada.
HSE (1988) The Tolerability of Risk from Nuclear Power Stations. UK Health and Safety Executive (HSE)
Hinrichsen K (2001) Critical appraisal of the meteorological basis used in (German) General Administrative Regulations (re: dispersion coefficients for airborne releases of NPPs)
See Annex D page 9: Radiation Biological Opinion. In http://www.strahlentelex.de/_03_Hauptgutachten_Stevenson–vollstaendig.pdf (in German)
Hodgson A, Scott JE, Fell TP, Harrison J. (2005) Radiation doses from the consumption of Cardiff Bay flounder containing OBT. J Radiol Prot. 2005; 25: 149-157.
Ichimasa M (1995) Overview of the 1994 chronic HT release experiment at Chalk River. Fusion Tech. 28:840-5.
ICRP Publication 30. (1979) Limits for Intakes of Radionuclides by Workers. Part 1. Annals of the ICRP volume 2, no.3/4.
Inoue Y et al (1993) Uptake of atmospheric tritium by market foods. Fusion Technology 21 pp 494-499.
IRSN (2010a).Sources of production and management of tritium produced by nuclear plants.Institute de Radioprotection etSureteNucleaire. Fonteney-aux-Roses, Paris France http://www.irsn.fr/FR/Actualites_presse/Actualites/Pages/20100709_rapports_IRSN_etat_connaissances_tritium.aspx
IRSN (2010b).Tritium in the Environment – Review of the IRSN.Institute de Radioprotection et SureteNucleaire. Fonteney-aux-Roses, Paris France. http://www.irsn.fr/FR/Actualites_presse/Actualites/Pages/20100709_rapports_IRSN_etat_connaissances_tritium.aspx
IRSN (2010c).Tritium in the Environment – A View from the IRSN on the key issues and avenues of research and development. Institute de Radioprotection etSureteNucleaire. Fonteney-aux-Roses, Paris France http://www.irsn.fr/FR/Actualites_presse/Actualites/Pages/20100709_rapports_IRSN_etat_connaissances_tritium.aspx
IRSN (2010d).Elements of reflection on the health risk posed by tritiumInstitute de Radioprotection etSûretéNucléaire. Fontenay-aux-Roses, Paris France http://www.irsn.fr/FR/Actualites_presse/Actualites/Pages/20100709_rapports_IRSN_etat_connaissances_tritium.aspx
IRSN (2010e).Tritium: Limits of releases and impact.Institute de Radioprotection et SureteNucleaire. Fonteney-aux-Roses, Paris France http://www.irsn.fr/FR/Actualites_presse/Actualites/Pages/20100709_rapports_IRSN_etat_connaissances_tritium.aspx
Radioactive Isotopes Examples
IRSN (2010f).Tritium and OSPAR. Institute de Radioprotection et SureteNucleaire. Fonteney-aux-Roses, Paris France http://www.irsn.fr/FR/Actualites_presse/Actualites/Pages/20100709_rapports_IRSN_etat_connaissances_tritium.aspx
Jaworowski K. (1982) Natural and man-made radionuclides in global atmosphere. IAEA Bulletin. 1982; 24 (2): 35-38.
Kaatsch P et al (2008) Leukaemia in young children living in the vicinity of German nuclear power plants. Int J Cancer.122(4) pp 721-6.
Kim et al (2013) Organically bound tritium (OBT) in soil at different depths around Chalk River Laboratories (CRL), Canada AECLNucl. Rev. (2013), pp. 17–26.
Kim SB, Bagman N, Davis PA.(2013 a) Current understanding of organicallyboundtritium (OBT) in the environment.J Environ Radioact. 2013 Dec;126:83-91.Table 3.
Kim SB, J. Roche (2012) Empirical insights and considerations for the OBT inter-laboratory comparison of environmental samples J. Environ. Radioact., 122 (2012), pp. 79–85
Kirchner G. A new hazard index for the determination of risk potentials of radioactive waste.J Environmental Radioactivity.1990; 11: 71-95.
Lane et al (2013) Radiation Exposure and Cancer Incidence (1990 to 2008) around Nuclear Power Plants in Ontario, Canada, Journal of Environmental Protection, Vol.4 No.9, September 2013.
Laurier D et al (2008) Epidemiological studies of leukaemia in children and young adults around nuclear facilities: a crit
Laurier D, Bard D (1999) Epidemiologic studies of leukemia among persons under 25 years of age living near nuclear sites. Epidemiol Rev 21(2):188-206.
Little MP, Wakeford R. Systematic review of epidemiological studies of exposure to tritium.JRadiol Prot. 2008; 28: 9-33.
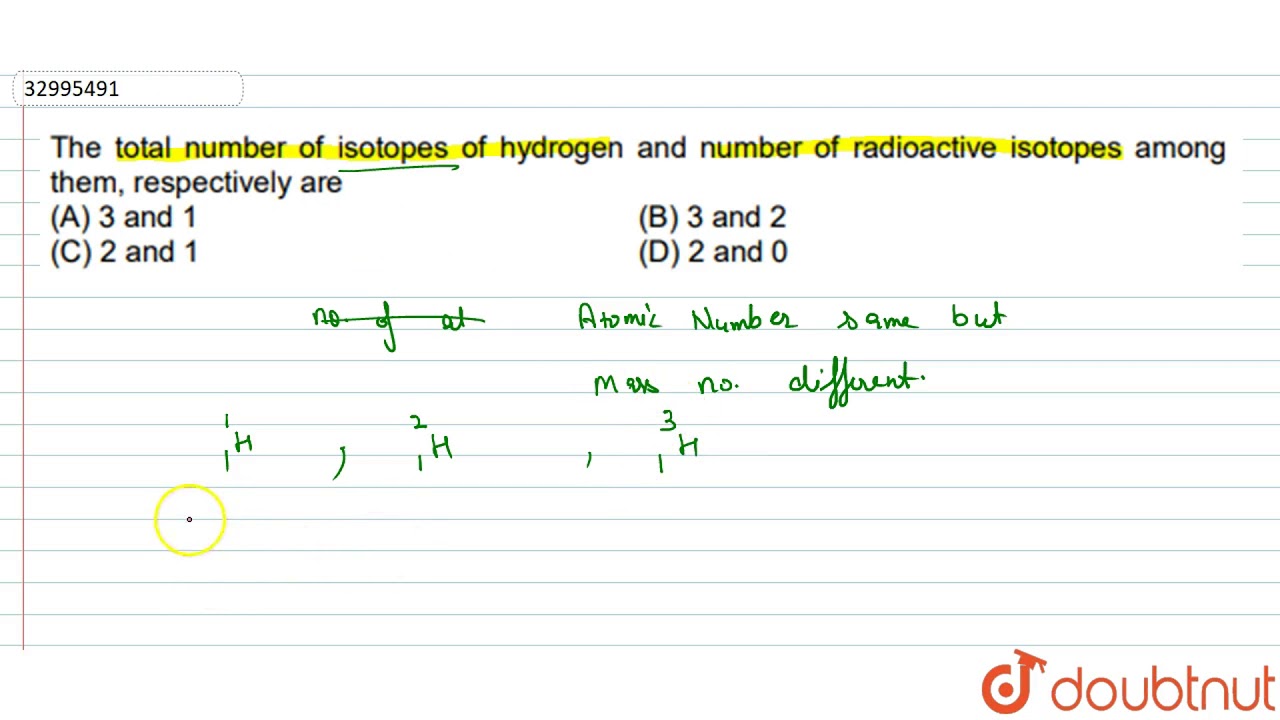
Makhijani A, Smith B, and Thorne MC. Science for the vulnerable: setting radiation and multiple exposure environmental health standards to protect those most at risk (chapter 7 on tritium). 2006. Available from: http://www.ieer.org/campaign/report.pdf
Melintescu A, Galeriu D, Takeda H. Reassessment of tritium dose coefficients for the general public. Radiation Protection Dosimetry. 15 June 2007: 1–5.
ODWAC (2009) Report and Advice on the Ontario Drinking Water Quality Standard for Tritium. Ontario Drinking Water Advisory Council http://www.odwac.gov.on.ca/reports/minister_reports.htm
The Radioactive Isotope Of Hydrogen Is
Okada S, Momoshima N. (1993) Overview of tritium: characteristics, sources, and problems. Health Physics. 1993; 65: 595-609.
Osborne R (2002) Tritium in the Canadian Environment: Levels and Health Effects. Report RSP-0153-1. Prepared for the Canadian Nuclear Safety Commission under CNSC contract no. 87055-01-0184 by Ranasara Consultants and Richard Osborne)
Rodgers DW. Tritium dynamics in mice exposed to tritiated water and diet. Health Physics.1992; 63: 331-337.
Shrader-Frechette K (1985) Risk Analysis and Scientific Method: Methodological and Ethical Problems with Evaluating Societal Hazards.D Reidel Publishing Co. Bpstpn US https://www.amazon.com/Risk-Analysis-Scientific-Method-Methodological/dp/902771844X#reader_902771844X
Spix C et al (2008) Case-control study on childhood cancer in the vicinity of nuclear power plants in Germany 1980 – 2003. Eur J Cancer. Jan; 44(2) pp 275-84.
Thompson PA et al (2015) Levels of tritium in soils and vegetation near Canadian nuclear facilities releasing tritium to the atmosphere: implications for environmental models. Journal of Environmental RadioactivityVolume 140, February 2015, Pages 105–113 http://www.sciencedirect.com/science/article/pii/S0265931X14003294
Trivedi L et al (1997) Dose Contribution from Metabolised OBT after Acute Tritium Water Intakes in Humans. Health Physics Vol 33 No 4. pp 579 – 586.
United Nations Scientific Committee on the Effects of Atomic Radiation (UNSCEAR).Sources and effects of ionizing radiation. Annex A: sources. New York: United Nations; 2006.
Uses Of Hydrogen Isotopes
Wanigaratne, E. Holowaty, H. Jiang, MSc, T. A. Norwood, M. A. Pietrusiak, P. Brown (2013) Estimating cancer risk in relation to tritium exposure from routine operation of a nuclear-generating station in Pickering, Ontario Chronic Diseases and Injuries in Canada, Vol 33, No 4, September 2013.
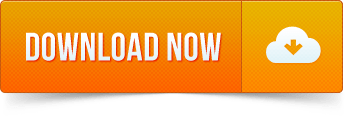